I. INTRODUCTION
Polymeric materials are widely used in various areas of nuclear power plants, such as wire cladding and packaging, among others. Structural changes of polymers can occur depending on radiation–irradiation conditions and polymer types. These structural changes cause the changes in the mechanical properties of polymers by cross-linking or oxidative degradation. Changes in the properties of the polymer materials are often related to changes in the free-volume hole radii.
Positron annihilation lifetime (PAL) techniques have been widely used in studying the defects of materials in the last two decades. PAL can be used as a powerful tool to measure the existence of free–volume holes radii R with a range of 2–20 Å in polymers. Determining the free volume plays an important role in characterizing polymers [1-3].
PAL provides unique information about free-volume size and concentration in polymers. PAL is defined as the time difference between the birth g-quantum of the β+-decay in the source and one of the annihilation g-quanta with an energy of 0.511 MeV. In 22Na source, photons with the energy of 1.27 MeV (start signal) are emitted simultaneously with the positrons. Thus, the time interval between the detection of photons of 1.27 MeV and 0.511 MeV can be detected from the experiments, as shown in Fig. 1.
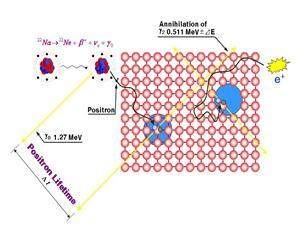
Figure 2 shows the schematic of the formation and annihilation of positronium (Ps) in polymers. The positrons generated in the β+-decay reaction have a broad kinetic energy distribution of up to 540 keV. When positrons are injected to a polymer, the former form positronium (Ps) in two states, namely, 75% as ortho-positroniums (o-Ps) and 25% as para-positronium (p-Ps). The hydrogen-like bound state p-Ps annihilates with a lifetime of the order of 0.125 ns (τ1 component) and decays with emissions of two photons. The spin-forbidden annihilation of the o-Ps decays after approximately 140 ns. The o-Ps annihilation may be enhanced if it is able to interact with other electrons and the spin exchange or pick-off annihilation occurs, thus reducing the lifetime to approximately 1 ns to 5 ns (τ3 component). Positrons that do not form Ps have a lifetime of approximately 0.4 ns (τ2 component) through interaction with the outer molecules with which they collide [4-6]. In the measurement of polymers by PAL, three states of positrons are characterized by lifetimes τ1, τ2 and τ3: anti-spin parallel p-PS with τ1 at approximately 125 ps, a free positron with τ2 at approximately 450 ps, and spin parallel o-Ps with τ3 at approximately 1 ns to 10 ns. In this study, the positron annihilation technique was used to measure the lifetime of a Ps atom. Among them, the third lifetime components the third lifetime component (τ3, o-Ps) is a function of the electron density at the annihilation site. Accordingly, the o-Ps lifetime has a strong correlation with the size of the free-volume holes, that is, as the holes become smaller, the o-Ps lifetime decreases. Therefore, the information of free-volume structures in materials can be obtained by PAL spectra.
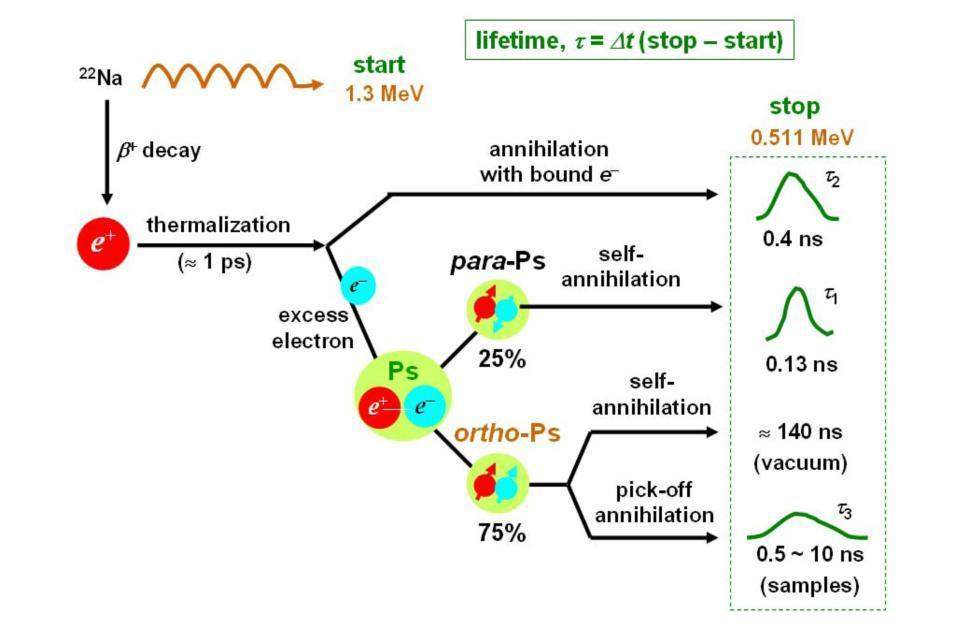
II. EXPERIMENTAL
A. Polymeric material samples
The polymer samples studied studied were nitrile butadiene rubber (NBR), chloroprene rubber (CR), and ethylene propylene diene monomer (EPDM) rubber. These materials are widely used for cable jacketing and insulation. The polymer materials were irradiated with 25 Gy/h in three conditions (50, 100 and 500 Gy) and 5 kGy/h in six conditions (5, 50, 200, 500, 800, and 1 000 kGy) at room temperature with 60Co gamma-ray sources. In total, 10 pairs of samples per polymer type were used in the PAL experiment, including non-irradiated samples. The high dose rate was chosen to identify the irradiation defect. Each pair of similar samples was irradiated for a calculated time and distance to achieve the required absorbed dose. Irradiation process with gamma rays was performed at the Korea Atomic Energy Research Institute – Advanced Radiation Technology Institute (KAERI-ARTI) in Jeongup, Republic of Korea. A high-level irradiation device (5 kGy/h at 85 cm distance between sample and source of 290 kCi) and a low-level irradiation device (25 Gy/h at 95 cm distance between sample and source of 1.77 kCi) were used based on the absorbed dose. Polymer samples were cut into two pieces with a size of 10 mm×10 mm×1 mm.
B. PAL spectroscopy
A conventional fast-fast coincidence system in KAERI was used to measure the lifetime spectra. This system consists of scintillators, a photomultiplier tube (PMT), a constant fraction differential discriminator (CFDD), time-to-amplitude converter (TAC), and multi-channel analyzer (MCA). The detectors are composed of barium fluoride (BaF2) scintillators coupled to fast photomultiplier tubes. Fig. 3 shows the components of the equipment used to measure the positron lifetimes. This experimental arrangement is called a fast-fast coincidence setup because the time measurement and the energy selection are performed in a fast channel. Fig. 4 shows the PA system and polymeric samples used in this study.
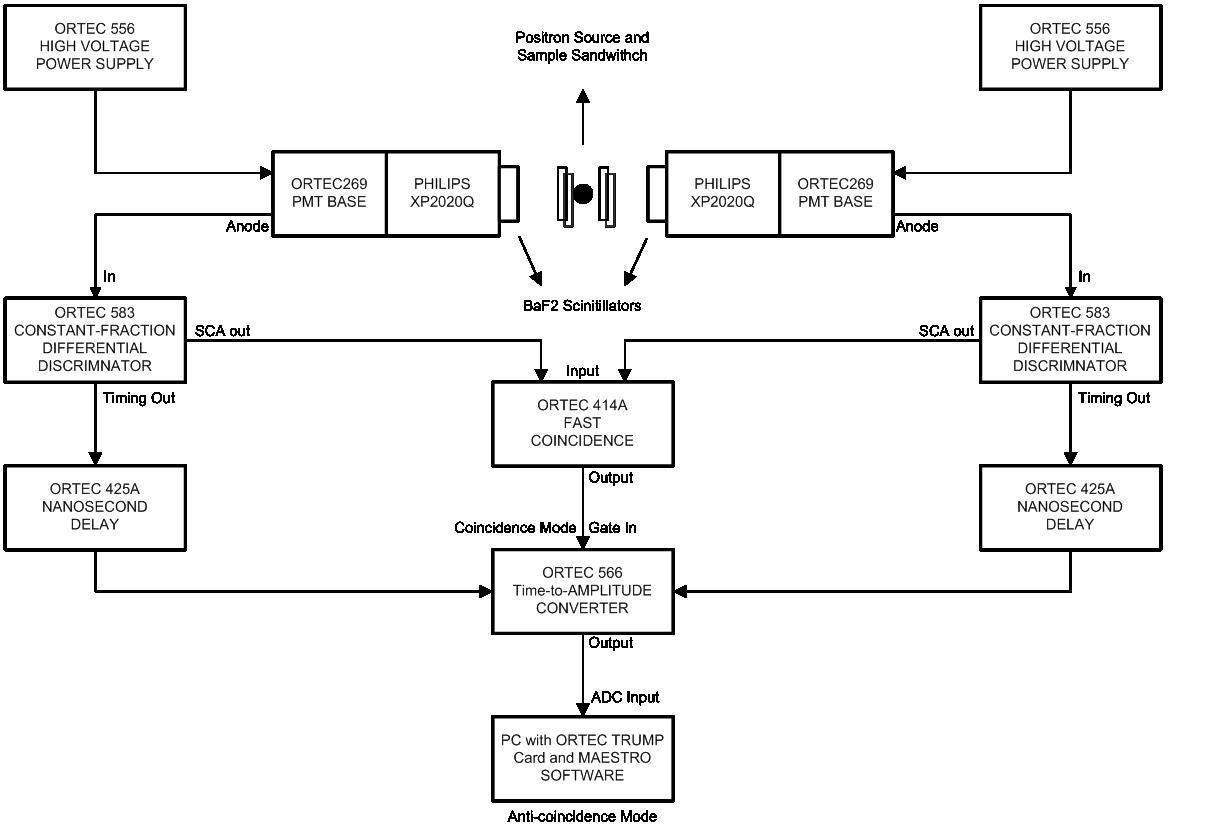
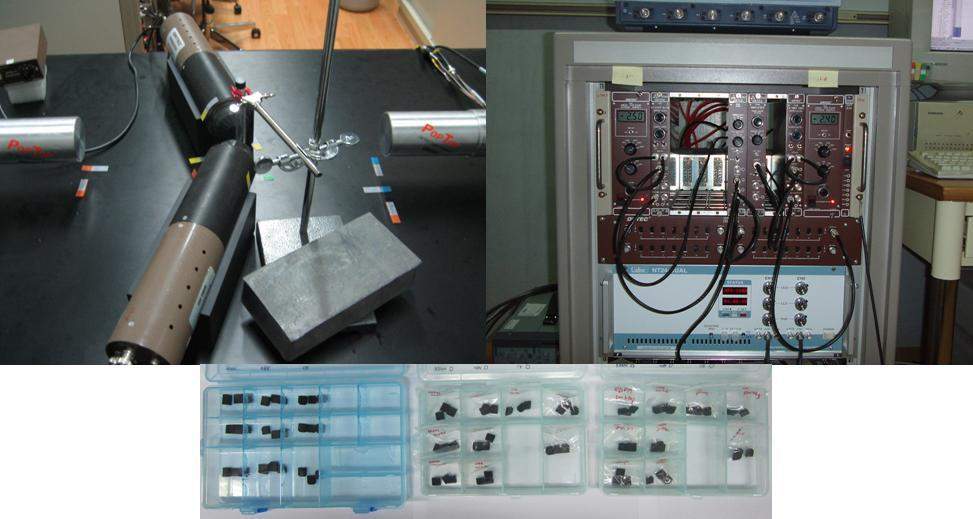
The instrumental time resolution of the system is 250 ps of the full width at half maximum (FWHM). The positron sources used for the measurement consist of 22Na sandwiched between two Ni foils. The equipment is kept in a room at a constant temperature (20 ± 1) °C to reduce the electronic drift. Through PAL, we can measure the time difference between the appearance appearances s of two quanta quanta (start and stop gamma ray). The measurement for each sample was continued for approximately 22 h, which corresponds to total counts of at least 2106. We applied the PAL fit program from the RISΦ laboratory to analyze the measured spectra [7].
C. Positronium in polymers
In the measurement of PAL in polymers, the three states of positron are p-Ps (τ1 approximately 125 ps), free positron (τ2 approximately 450 ps), and o-Ps (τ3 approximately 1 ns to 10 ns). The positron lifetime of o-Ps reflects the information of free volume in polymers. Fig. 5 shows the concept of free volume in polymers.
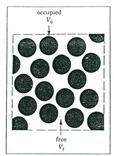
The free volume holes can be calculated from the lifetime τ3 in a spherical approximation of the Tao-Eldrup [4, 8] model as
where R0 = R + ΔR and ΔR = 0.166 nm is the thickness of the homogeneous electron layer as empirical parameter in which the positron in o-Ps annihilates via the so-called pick-off process [9]. The lifetime τ3 and radius R are expressed in ns and Å, respectively. The fractional free volume (FFV) parameter Vf could be estimated through the lifetime measurements of polymers. FFV(f) is defined as
where V is the total macroscopic volume of the polymer, V0 is the volume occupied by molecules, and Vf is the free volume of the polymer. Free volume size Vf in nm3 and FFV (%) are calculated as Eqs. 3 and 4, respectively.
where A is the constant correction factor (= 0.016), and I3 is the relative intensity of the o-Ps lifetime component.
III. RESULTS AND DISCUSSION
The experimental data of the three component positron lifetimes and the intensity of o-Ps component I3 for EPDM, NBR, and CR are shown in Figs. 6 and 7, respectively. The variations of positron lifetime based on the absorbed dose are apparently not large. The positron lifetime τ3 is related to the size of the free volume the free volume in polymers. The gamma-ray exposure within these dose ranges does not seem to result in significant changes in the free volume size in polymer samples. By contrast, the relative intensity of τ3 decreased based on the absorbed dose. This result means that the relative fraction of the free volume in samples is decreased. Our experiments indicate that Ps lifetime is not changed while its intensity decreases with the absorbed dose.
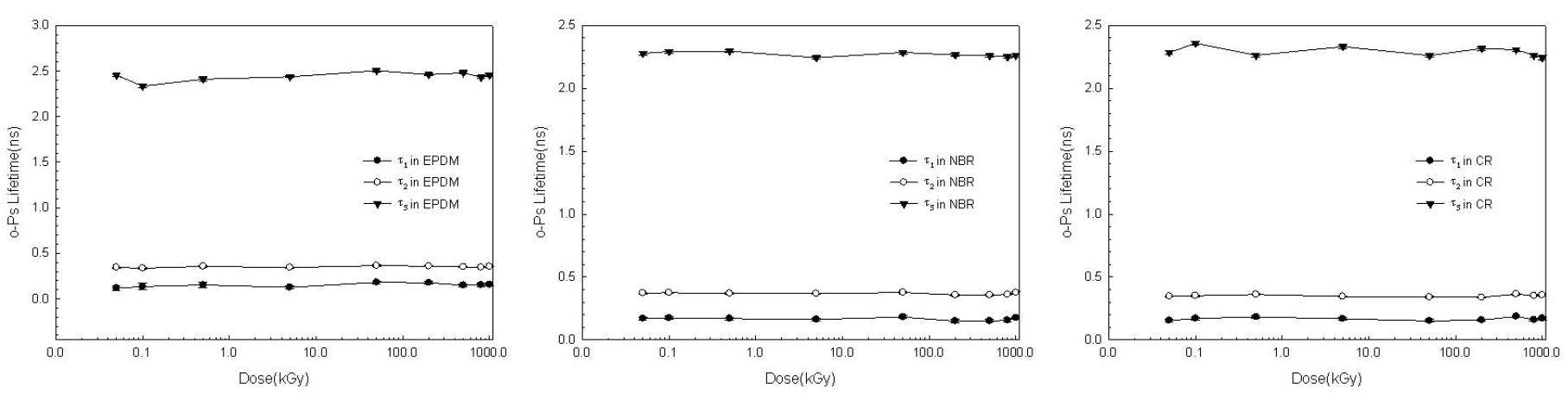
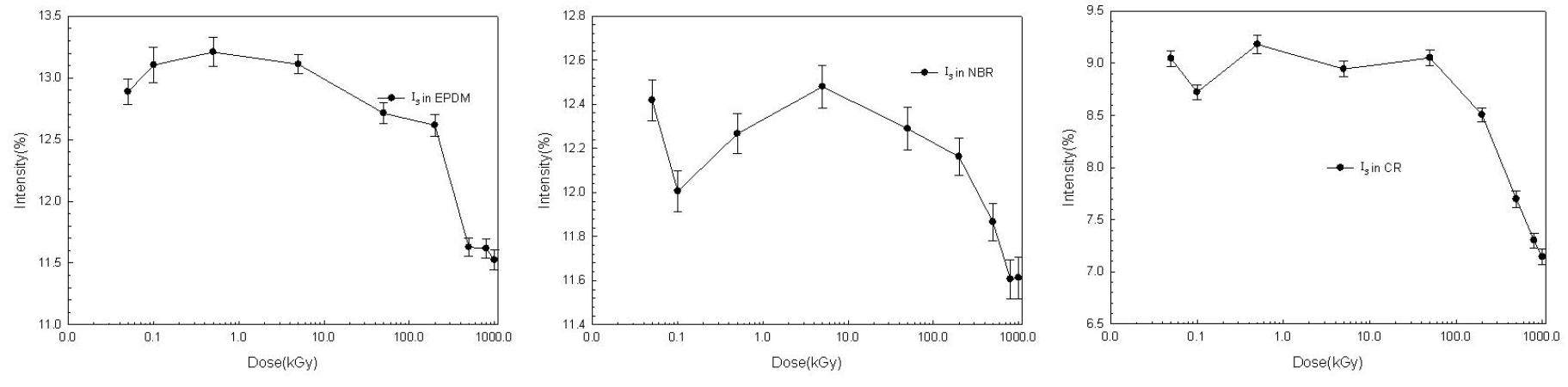
Based on Eq. 1, the mean radii of the free volume in samples before irradiation were calculated as 3.260 Å (EPDM), 3.073 Å (NBR), and 3.074 Å (CR). Moreover, the variation of the mean radii was not large even after gamma-ray irradiation. Vf and I3 have to be explicitly considered to obtain a reasonable estimate for the total free volume in the polymer samples. Thus, we calculated the relative FFV by using Eq. 4. The results are shown in Fig. 8.
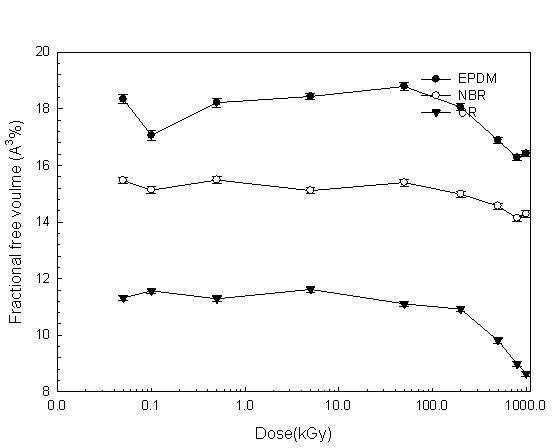
Cross-linking and degradation could occur when a polymer is irradiated. Gamma-ray irradiation results in decreased FFV of polymers. Our experiments indicate that the relative Ps lifetime intensity decreases with the increase of absorbed dose. This result means that irradiation affects the structure of polymers. CR polymer materials demonstrate relatively low FFV. More cross-linking by radiation was estimated to have occurred. By contrast, less decrease occurred based on the irradiation in NBR samples. These properties have been discussed in the published papers [10-12].
IV. DISCUSSION AND CONCLUSION
We applied PAL spectroscopy on EPDM, NBR, and CR polymer samples to the measurement to the measurement of relative fraction of free-volume hole. PAL should not be regarded as an absolute but rather a relative measurement technique. Thus, we calculated a relatively FFV value from the Tao-Eldrup model as a characteristic measure. We confirmed FFV decrease after gamma-ray irradiation. These results were verified through PAL measurement, from which we identified the feasibility of PAL for polymer analysis. Ps was determined to be a suitable probe for characterizing the free-volume structure of the size distribution and the sample density.