I. INTRODUCTION
99mTc, one of the most-used radiopharmaceuticals, has physical advantages for diagnosis. Its 6-h half-life and negligible beta-like radiation permit the clinician to administer several millicuries with relatively low radiation burdens. Furthermore, its exclusive emission of 140-keV photon is close to ideal for camera imaging technology. These characteristics make 99mTc widely used with various ligands. The more 99mTc are used for radiopharmaceuticals, the more adequate assessment of the internal exposure is needed for not only patient but also medical worker. The patient internal exposure by an intravenous injection has been assessed relatively well with the reports of ICRP or MIRD. In 2007, ICRP published the report 'Radiation Dose to Patients from Radiopharmaceuticals(ICRP 106 [1])’ to provide biokinetic and dosimetric models for 33 radiopharmaceuticals. The systemic model and dose coefficients recommended in ICRP publication 53 [2], 80 [3], 106 facilitate the assessments of internal exposures for patients receiving 99mTc via intravenous injection. In case of medical workers, on the other hand, there are few studies about the internal exposure caused by inhalation and ingestion although significant exposures are predicted. The dose coefficients of 99mTc in ICRP publication 68 [4] are only available information for worker. However, the dose coefficients are not enough for medical field where various 99mTc-labelled agents are treated because the behavior after uptake is dependent on the type of ligand. In addition, the recalculations are needed based on the new recommendations of ICRP publication 103 and OIR report [5] which describes the more reliable biokinetic models for occupational intakes. For these reason, the purpose of this study is to calculate intake retention and excretion function and dose conversion coefficients which can be used for the assessment of internal exposure. The two most frequently used 99mTc-labelled compounds were selected; 99mTc-MDP, Pertechnetate, used in bone scan, thyroid scan, respectively. This report provides the fitting coefficients of their whole-body retention and urinary excretion functions with the committed dose conversion coefficients.
II. MATERIALS AND METHODS
A. Selection of 99mTc radiopharmaceuticals
Korea Society of Nuclear Medicine listed the nuclear medicine scans with the number of times being executed during recent 51 years in South Korea [6]. It notes that the bone scans using 99mTc-phosphonates such as 99mTc-MDP, 99mTc-HDP, 99mTc-HDEP, 99mTc-DDP are most executed since 1988. Therefore, it could be expected that the most internal exposures of medical workers occur by the 99mTc-phosphonates. Furthermore, the pertechnetates used for thyroid scan could bring out considerable internal exposures because thyroid scans with pertechnetates are very common. The assessment of pertechnetate is especially important because the 99mTc is generated as the form of pertechnetate (
B. Biokinetic models for 99mTc-labelled compounds
The actual metabolism in human body including retention and excretion of materials is too complex to descript real physiological behaviors. To depict the metabolism practically, simple compartment models have been used to calculate the retention and excretion in human body. In 2006, ICRP recommended the HATM(Human Alimentary Tract Model, publication 100 [7]) which could depict the behavior of ingested materials before uptake to blood. The HATM could describe more realistic behaviors of ingested materials in all alimentary tract regions including oral cavity, oesophagus, stomach, small intestine, right colon, left colon and rectosigmoid. This recent HATM was adopted in our calculations. For inhaled materials, the HRTM(Human Respiratory Tract Model) recommended in ICRP publication 66 [8] could depict its deposition and absorption in respiratory tract. However, the HRTM was revised in the OIR(Occupational Intakes of Radionuclides) draft report which reflected the new recommendations in ICRP 103. The revised HRTM describes the behavior after inhalation more realistically and simply. Therefore, we used the revised HRTM in OIR for more reliable results other than original HRTM. In this section, the more detail review of changes in revised HRTM and the systemic models which depict the behavior after uptake to blood are included.
1. Systemic models
The physiological behavior of 99mTc-compound after absorption to blood is different in type of ligand. It is clear the different systemic models are needed according to ligand. At first, the systemic model of pertechnetate has been relatively well established because it is the most readily available chemical form and the starting point for technetium chemistry. In addition, the revised systemic model of technetium provided in OIR has been developed based on the pertechnetate experimental results. Therefore, it could be directly adopted for occupational intake of pertechnetate. The systemic models of pertechnetate depicted in OIR part 2 is as in the following [12]; The initial distribution of pertechnetate is similar to that of inorganic iodide. Pertechnetate absorbed to blood is selectively concentrated in the thyroid, salivary glands, and stomach wall. In contrast to iodide, pertechnetate trapped by the thyroid is not organically bound in the thyroid but is largely released back to blood over a period of hours. Compartments representing the thyroid, salivary glands, stomach wall, and right colon wall are added to the model because they have been identified in human or animal studies as important repositories for pertechnetate. The bone, kidneys, liver, thyroid, and other soft tissues are each divided into multiple compartments representing different phases of retention and, in the case of bone, also different types of tissue. The structure of the biokinetic model for systemic technetium used in OIR is shown in Fig. 1.
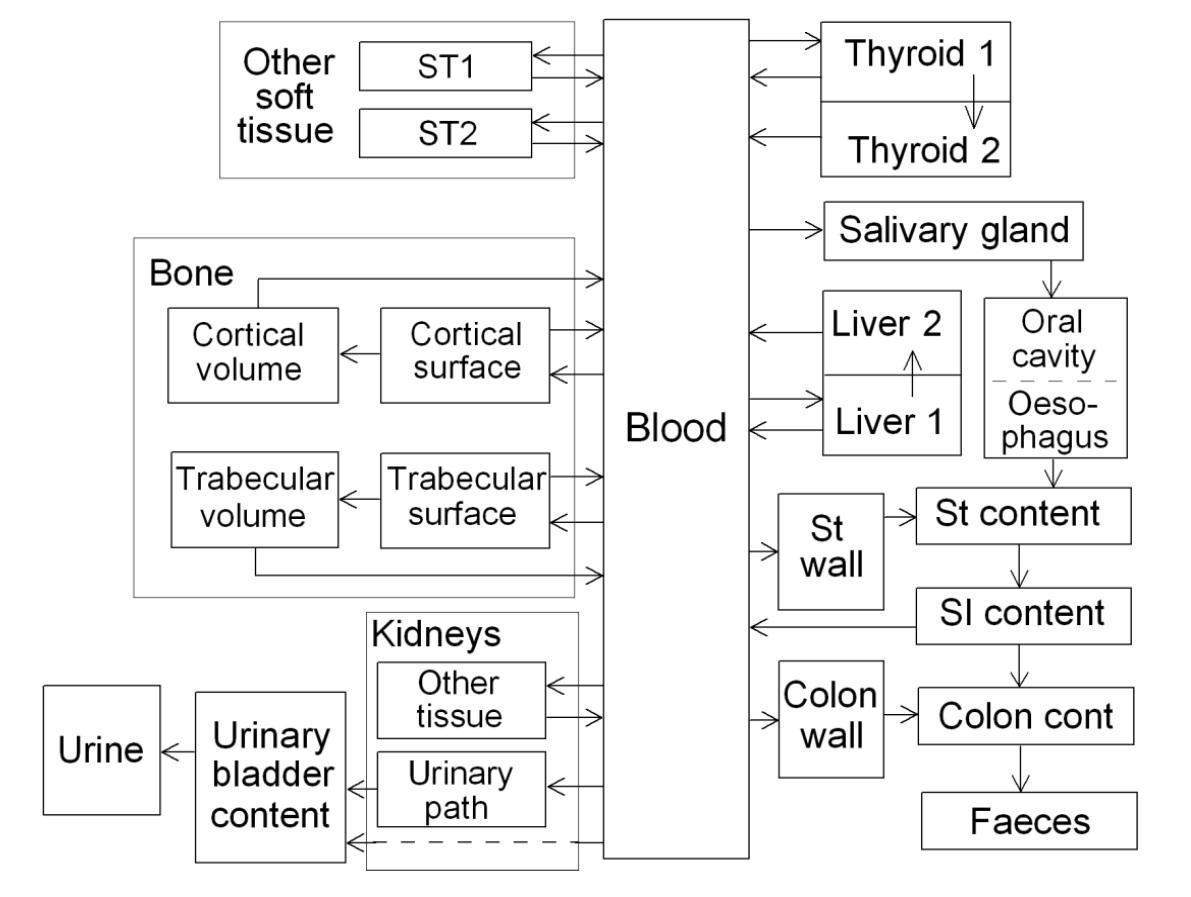
In case of 99mTc-phosphonates, the situation is a little different. The systemic model recommended in ICRP publication 53 had been developed not for workers but for patients. Nevertheless, we used the model to predict the behavior of material absorbed to blood. It is based on the assumption that the behaviors after uptake to blood are equal regardless of intake routes. In addition, the various kinds of 99mTc-phosphonates used for bone imaging have the sufficient biokinetic behavior to justify the use of a common biokinetic model. Therefore, the biokinetic model of 99mTc-phosphonates described in ICRP publication 53 was used and it is shown in Fig. 2. The main uptake is in bone, with a further small uptake in kidneys, and the excretion is via the renal system. The transfer rates were calculated using leaving fractions from a transfer compartment and biological half-life.
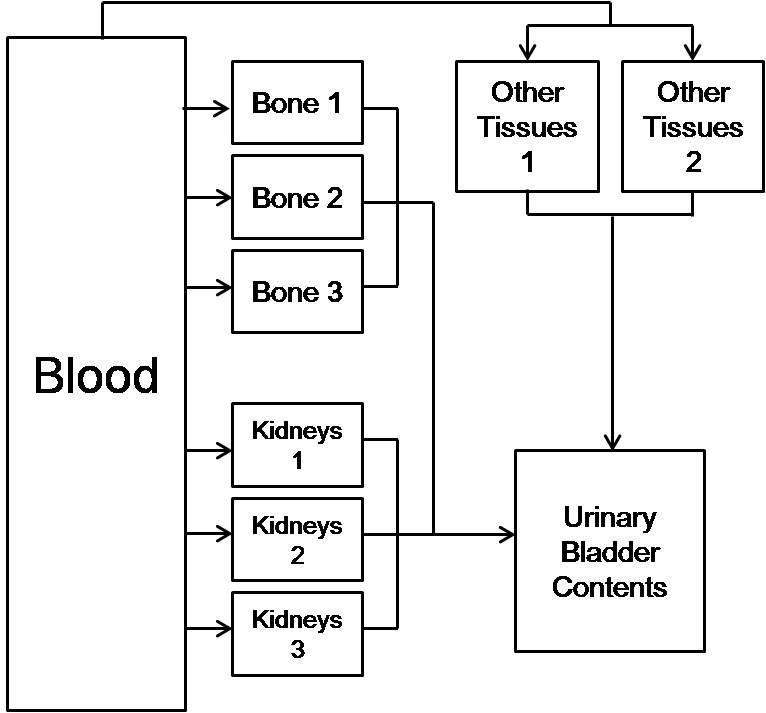
2. Changes of the human respiratory tract model.
Original HRTM: The HRTM described in Publication 66 (ICRP, 1994a) was applied to calculate inhalation dose coefficients and bioassay functions in recent reports. As in the original version of the HRTM, the respiratory tract is treated as two tissues: the extrathoracic regions (ET) and the thoracic regions (TH). The sub-division of these tissues into regions was based mainly on differences in sensitivity to radiation. The thoracic regions are bronchial (BB), bronchiolar (bb), alveolar-interstitial (AI); and the thoracic lymph nodes, LNTH. The extrathoracic regions are the anterior nasal passage, ET1; the posterior nasal passages, pharynx and larynx, ET2; and the extrathoracic lymph nodes LNET.
Deposition: No changes are made in revised HRTM to the original HRTM of deposition model for aerosols, except for the distribution of the deposit in the ET airways between regions ET1 and ET2. In ICRP publication 66 the same deposition fraction was assumed for ET1 and ET2 although actual deposit amount of ET1 was more than ET2. In OIR, however, more realistic fractional deposition in extrathoracic region is adopted because the more realistic transfer rate is available[13](see below).
Particle transport: The original HRTM was revised for simpler and more realistic particle transport in OIR report. The important change is the transfer rate from ET1 to ET2. While the transfer was not allowed in original HRTM, it is assumed that material deposited in ET1 is cleared at a rate of 2.1×10-1 on the basis of recent data; about one-third, by nose blowing and two-thirds by transfer to ET2. This change will increase systemic uptake in ET2 and the alimentary tract. In case of the slow clearance in the revised HRTM, it occurs only in the bronchiolar(bb) region. The rate from bb to BB was decreased by a factor of ten instead of omission of BB2, bb2 compartments. In addition, the changes of transfer rate in alveolar-interstitial region show that greater long term retention in the AI region is assumed.
Absorption to blood: Absorption to blood of materials deposited has been classified according to absorption speed; F, M, S. While the absorption parameter values of original HRTM were not based on experimental data, ICRP recommended the more realistic values based on recent data in revised HRTM. In OIR report, however, the material-specific parameter values are offered where sufficient information is available. For this reason, more reliable assessment could be made when the material information is known. The material-specific parameters of pertechnetate have been offered with type 'F’ in OIR part 2 whereas 99mTc-phosphonate has been assigned to type 'M’ as 'unspecified forms’. AMAD (Activity Median Aerodynamic Diameter) was considered as 5 μm recommended for workplace exposures.
3. Calculation of retention functions, excretion functions and dose coefficients
The intake retention and excretion functions can be calculated using the algorithm proposed by Birchall and james [9] with the transfer rate. The algorithm first transforms the rate matrix into a new matrix [A]. If rji is the transfer rate from compartment i to j and aij is the value of the elements of the matrix [A], respectively, then
and
.
Once the matrix [A] is formed, the amount in compartment i at any subsequent time t could be calculated by
where e[A] is the exponential of the matrix [A], and qi(0) is the column vector of initial amounts in each compartment i when the unit activity, 1Bq, is taken into the body. For a biokinetic system consisting of parent and decay products [10], the matrix [A] become
where M, λM are the rate matrix and decay rate of the parent, and D, λD are the rate matrix and decay rate of decay product, respectively. In this study, parent and decay product are 99mTc and 99Tc. The retention and excretion function were calculated every hour up to 20 times the half-life of 99mTc using MATLAB. For using in bioassay the intake retention function or excretion function, m(t), can be written
where ai, λi are fitting coefficients of i-th term. The fitting was conducted using ORIGIN program. After the intake retention and excretion functions are obtained, we determined the intake amount of radionuclide, I, by
where M is measured quantity.
For calculations of dose coefficients SAF values for photons calculated by Cristy and Eckerman were used [11]. However, in some cases, the SAF values were surrogated for suitable regions because the results were not available for all source and target regions of HATM. For example, SAFs for ULI of Cristy and Eckerman were used for the right colon and left colon, SAFs for LLI were used for rectosigmoid colon [13]. In future ICRP reports, however, the results calculated using the reference voxel phantoms will be published. The SEE values table was obtained by SEECAL 2.0. The number of nuclear transformation that have occurred up to 50 years in compartment i, U(50), can be calculated by
the constant, c, relates contents to nuclear transformations in the desired units.
Dose coefficients could be calculated using the U(50) and SEE values with tissue weighting factor, wT, in ICRP publication 103. The calculation procedures were achieved by MATLAB.
III. RESULTS AND DISCUSSION
The fitting coefficients of whole-body intake retention functions for 99mTc-phosphonate and pertechnetate are shown in Table 1 with the intake routes. The adjusted coefficients of determination, R2, have verified that the functions fitted by only two exponential terms are statistically significant. Since the whole-body retention curve was relatively simple shape as decreasing function, the fitting coefficients could be obtained easily.
Intake Route | Parameter | α1 | λ1 | α2 | λ2 | R2 | |
---|---|---|---|---|---|---|---|
99mTc-phosphonate | Inhalation | Type M | 0.84121 | 3.06527 | -0.02159 | 1.78027 | 1.0000 |
Ingestion | 0.2 | 1.00081 | 4.03283 | 0.03335 | 1.29644 | 0.99877 | |
Pertechnetate | Inhalation | Type F | 0.91251 | 3.24075 | -0.09317 | 3.24132 | 0.99996 |
Ingestion | 0.9 | 1.00877 | 3.14504 | 0.0028 | 0.90491 | 0.9999 |
For the urine excretion function, however, more terms were needed to have reliable statistical explanation ability. As shown in Table 2, the daily urinary excretion function was expressed by four exponential terms because the urinary excretion has several patterns with time. Table 3 shows the committed dose conversion coefficients that were calculated based on the OIR models.
Intake Route | Parameter | α1 | λ1 | α2 | λ2 | α3 | λ3 | α4 | λ4 | R2 | |
---|---|---|---|---|---|---|---|---|---|---|---|
99mTc-phosphonate | Inhalation | Type M | 1.82824 | 6.03401 | -1.20666 | 2.8036 | 1.2807 | 2.8034 | -1.90202 | 5.89044 | 0.99906 |
Ingestion | 0.2 | -0.10173 | 2.69365 | 0.51893 | 2.69532 | -2.85272 | 10.0277 | 2.4375 | 11.63239 | 0.99922 | |
Pertechnetate | Inhalation | Type F | -0.21132 | 4.14412 | 0.18891 | 2.79974 | -3.19384 | 13.15714 | 3.21622 | 13.17308 | 0.99999 |
Ingestion | 0.9 | -0.25268 | 2.18157 | 0.41867 | 2.30572 | -2.61826 | 8.50241 | 2.45247 | 8.9645 | 0.99995 |
Committed effective dose coefficients (Sv/Bq) | ||
---|---|---|
Inhalation, einh(50) | Ingestion, eing(50) | |
99mTc-phosphonate | 7.33×10-11 | 3.04×10-11 |
Pertechnetate | 6.71×10-11 | 7.41×10-11 |
The assessments of internal exposures of the medical workers who treat 99mTc radiopharmaceuticals could be conducted by whole-body counting or urinary sampling. Thus, the results of this report could be used to estimate the intake of radionuclides and committed effective dose for inhalation or ingestion.
IV. CONCLUSION
In this study, the intake retention and urinary excretion function were provided with fitting coefficients for the assessments of internal exposures for medical workers. The results carry an important meaning in that the revised compartment models based on recent experimental results were adopted. Furthermore, the dose coefficients in this paper may be used to estimate the internal dose caused by 99mTc exposure. Although the recalculation is needed after the new SAF values derived from the reference voxel phantom are published, we think the dose coefficients of this report are the best for now. Exposures by 99mTc have been considered one of the main intakes for medical field and its internal dosimetry needs the retention and excretion functions of this study. Therefore, the results of this study are expected to be useful to assess the internal exposure for medical workers.
Specific absorbed fractions of energy at various ages from internal photon sources. Oak Ridge National Laboratory Report
, 1987, TM-8381, V1.